In
Metazoa:Animal Minds and the Birth of Consciousness, author Peter Godfrey-Smith describes the
Hexactinellida, a group of sponges that construct hard parts made of silicon dioxide as a support for its soft tissue. In an
earlier post I had written briefly about amorphous varieties of silica. The Hexactinellidae's skeleton is made up of opal, denoted by the chemical formula SiO2.nH2O. Sponges put together their skeleton using a variety termed opal-A , the A indicating amorphous. Over geologic time the amorphous opal-A often transforms by expelling water and re configuring the geometry arrangement of silicon and oxygen atoms to opal-CT and chalcedony, both silicon dioxide varieties showing the first glimmer of a crystalline structure.
Hexactinellida are popularly called the glass sponges because of their transparent silica frame. The basic elements of this skeleton are tiny rods or spicules which are joined to form dagger, star or snowflake like shapes. These then group together to form a hard mesh that supports the soft tissue. Upon death, the silica skeleton disintegrates, leaving a carpet of spicules on the sea floor.
The sketches below are from Godfrey-Smith's book. They are drawings by Rebecca Gelernter of sponges collected on the Challenger expedition of the 1870's.
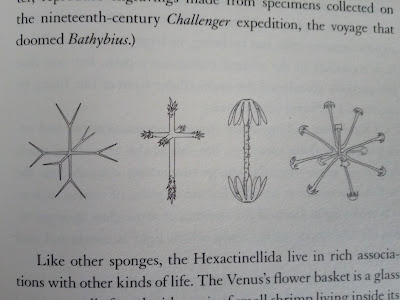
One fascinating function of these glass elements could be as collectors of light. Sponges often have colonies of photosynthetic organisms like diatoms living inside them. The speculation is that the glass channels light energy into the interior of the sponge body, which the diatoms use as a power source for photosynthesis.
Glass gardens on the sea floor is an evocative way to describe these sponge communities. And occasionally in geologic history these gardens have proliferated on a scale that is simply hard to imagine. Some time back I read a very interesting paper by Edward J. Matheson and Tracy D. Frank on Late Permian age (~260 million years old) sedimentary rocks deposited on the northwestern shores of the supercontinent Pangea. Different sedimentary rock types were deposited in this long lived basin. One distinct layer, termed the Tosi Chert, contains significant amounts of chalcedony and chert. A closer examination revealed that these two silicon dioxide minerals were derived from a siliceous sponge precursor.
Scattered through these Permian rocks are 'ghosts' of spicules. The Tosi Chert was once a glass sponge garden colonizing a gently sloping sea floor. It was staggering in scale. These sponge meadows extended over 75,000 sq km. To the east of these sponge habitats lay an arid Laurentian desert, Laurentia being the northern continent which had joined the southerly placed Gondwana to form the supercontinent Pangea. To the west was the subtropical epicontinental Phosphoria Sea. An epicontinental sea is a shallow sea that floods the interiors of continents during times of a global sea level high. Since siliceous sponges were the dominant benthos these depositional systems are called glass ramps, the latter term indicating a uniformly sloping sea bed. The paleogeographic map below shows the position and range of the 'spicule belt' (in orange) on the northwestern edge of Pangea. The pale pink area is the desert.
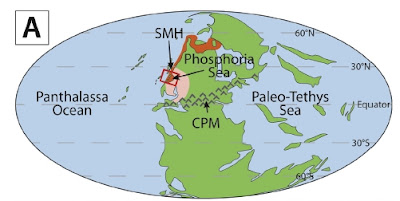
Source: An epeiric glass ramp: Permian low-latitude neritic siliceous sponge colonization and its novel preservation (Phosphoria Rock Complex) Edward J. Matheson and Tracy D. FrankThe Tosi sponge communities lived during a time of sea level rise. The sedimentary variation within the Tosi Chert indicates that sponges occupied environments ranging from subtidal settings to near shore tidal flats. In the open ocean subtidal regions the sediment was mostly sponge debris. Nearer to the shore the environments were more variable. Calcium carbonate mineralizing organsims such as molluscs lived in patchy zones. Abiogenic ooids formed in some areas. In other regions, currents transported quartz detritus from adjacent areas. Wind blown silt size mica and iron oxide particles sourced from the eastern deserts mixed with the biogenic sediment. Landward, in shallow ponds and depressions, layers of gypsum precipitated from saline waters.
These environments of deposition of the Tosi Member are depicted in the block graphic below.
Source: An
epeiric glass ramp: Permian low-latitude neritic siliceous sponge
colonization and its novel preservation (Phosphoria Rock Complex) Edward J. Matheson and Tracy D. Frank
These conditions persisted for hundreds of thousands of years. Eventually, sea level began to fall and the sponge communities began to die out. Calcareous biota replaced the silica sponges. The glass gardens were buried under layers of lime sediment.
Like an artist dismantling a patiently constructed exhibit of installation art, nature relentlessly ground up the delicate glass sponges and transformed them into rock. But this change took its own interesting route.
As sea level dropped, a mosaic of tidal flats and lagoons developed. In the arid climate, high rates of evaporation resulted in the development of hypersaline magnesium rich brines. These denser pools of water percolated downwards through the shallow buried silica rich sediment. The magnesium calcium carbonate mineral dolomite started precipitating within the sponge rich sediment. Along with dolomite, the calcium sulphate mineral gypsum formed at places.
The dolomite rich sediment then underwent another transformation. The opal skeletons of the sponges started dissolving. The released silica however did not diffuse away in to the open sea. Rather, the high amounts of released silica created zones of silica supersaturation within the pore spaces of the sediment resulting in the precipitation of chalcedony and chert. Silica got redistributed within the Tosi sediment package, first dissolving and then reprecipitating a few millimeters away. The new silica minerals were not spread evenly but formed compact masses giving the evolving rock a nodular appearance. Here and there the original shapes of the sponge spicules were preserved, although they were no longer made up of opal, having being replaced by chalecdony and chert.
The photomicrographs show examples of dolomite and silica nodule replacement of the original sponge skeletal debris. The pale area in the image to the left is a chert nodule with a diffuse boundary that gives way to a darker dolomite matrix. The image to the right shows a bioturbated dolomite rock with some chert replacement. Tiny lath shaped particles are ghosts of sponge spicules.
Source: An
epeiric glass ramp: Permian low-latitude neritic siliceous sponge
colonization and its novel preservation (Phosphoria Rock Complex) Edward J. Matheson and Tracy D. Frank
Today the Tosi Chert is not that attractive or spectacular rock to look at. It is a few meters thick, has a grey to red to purple color and is made up mainly of silica nodules and dolomite with minor amounts of quartz, anhydrite and gypsum. Layers of limestone, lithified from patchy molluscan and ooid sediment, interfinger with silica rich strata.
Calcium carbonate secreting organisms have been the most prolific biogenic sediment producers in Phanerozoic shallow marine settings. Siliceous sponges more commonly occur in deeper water and high latitude settings. Occasionally though, siliceous sponges did take over the shallow marine domain. The extensive Mid-Late Permian Pangean sponge belt is an example of such ecological opportunism, where silica rich sea water and nutrient availability resulted in prolific growth and persistence of sponge communities over vast areas of the northwestern Laurentian margin. Those majestic glass gardens, perhaps harboring photosynthetic symbionts are now gone, transformed to dull looking rock, but look closely and the ghosts of those long dead sponges are waiting to tell you their story.